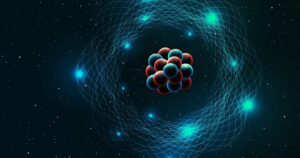
Two of the most well-known physicists of the day, Albert Einstein and Erwin Schrödinger, got into an argument on the nature of reality in 1935.
After performing the necessary calculations, Einstein concluded that the universe must be local and that no occurrence in one place can instantly affect another. However, Schrödinger had performed his own calculations and was aware that the fundamental concept of quantum mechanics was an odd relationship he called “entanglement,” which seemed to contradict Einstein’s rational premise of locality.
When two particles collide, which can result in their becoming entangled, their fates are intertwined. If you measure the orientation of one particle, for example, you might discover that its entangled companion (if and when it is measured) always faces the opposite way, regardless of where it is. Thus, it would appear like Einstein’s dictum that no influence can travel faster than light is being broken if a measurement in Beijing appeared to instantly alter an experiment in Brooklyn.
The extent of entanglement bothered Einstein (who later referred to it as “spooky”), and he challenged the still-developing theory of quantum mechanics for being inherently flawed. In response, Schrödinger defended the theory he had helped develop. But he understood Einstein’s aversion to entanglement. He acknowledged that it was “a little unsettling” because it appeared to let one experimenter “steer” an otherwise unreachable experiment.
Since then, physicists have mostly let go of that unease. They now realize that entanglement has no remote influence, something that Einstein and possibly Schrödinger himself had ignored. It can only disseminate information about that conclusion; it cannot physically bring about a certain outcome. The Nobel Prize-winning entanglement experiments of 2022 are now commonplace.
A flurry of theoretical and experimental research over the past few years has revealed an odd new face of the phenomenon—one that manifests itself not in pairs but rather in constellations of particles. A set of particles becomes entangled organically, creating a complex web of consequences. However, you can prevent the web from forming if you monitor the particles frequently enough and eliminate entanglement in the process. Three groups of theorists demonstrated in 2018 that these two states, web or no web, are similar to well-known states of matter like liquid and solid. The difference between web and no web, however, denotes a change in the structure of information rather than a change between various matter types.
One of the physicists who initially recognized the phenomena, Brian Skinner of Ohio State University, observed, “This is a phase transition in information.” “It’s where the properties in information—how information is shared between things—undergo a very abrupt change.”
More recently, a different set of teams made an effort to watch that phase change take place. In order to determine how measurements themselves impact the flow of information, they carried out a number of meta-experiments. Quantum computers were utilized in these studies to demonstrate that it is possible to strike a careful balance between the conflicting effects of entanglement and measurement. The finding of the transition has spurred a wave of investigation into the potential outcomes of the interaction between entanglement and measurement.
An Entangled Dessert
Over sticky toffee pudding at a restaurant in Oxford, England, one of the collaborations that stumbled onto the entanglement transition was formed. Skinner paid his friend Adam Nahum, a physicist working at the École Normale Supérieure in Paris, a visit in April 2018. They got into a long discussion on entanglement and information and found themselves arguing a fundamental issue.
First, a little look back. Imagine a pair of particles, A and B, each with a spin that can be measured as pointing up or down. This will help you to grasp what entanglement has to do with information. A measurement yields a random result, either up or down, because every particle starts off in a quantum superposition of up and down. Measurement of the particles is equivalent to flipping two coins if they are not entangled: Gaining the upper hand in one does not guarantee success in the other.
However, the two results will be connected if the particles are entangled. For instance, if you discover B pointing up, a measurement of A will show it pointing down. The two share an “oppositeness” that doesn’t live in each individual but rather between them; it has a fragrance of the nonlocality that both Einstein and Schrödinger found unsettling. This oppositeness has the effect of allowing you to learn about the other particle by measuring just one of them. “Measuring B first gave me some information about A,” explained Skinner. That lessens my lack of knowledge regarding the state of A.
The entanglement entropy is a measure of how much a measurement of B reduces your ignorance of A, and like any type of information, it is measured in bits. The primary measure of entanglement used by physicists to determine how much information about one object is stored nonlocally in the other is called entropy of entanglement. Entropy equals entanglement, therefore if there is none, measuring B tells us nothing about A. Because there is a lot of entanglement when the entropy is high, measuring B reveals a lot about A.
Skinner and Nahum advanced this reasoning by two during dessert. First, they made a chain as long as one could envision out of the pair of particles. They understood that entanglement would spread like the virus from one particle to the next in accordance with Schrödinger’s namesake equation, the quantum mechanical equivalent of F = ma. They also understood that by using the same formula, they could determine how firmly entanglement had taken hold: If entanglement entropy is high, the two sides of the chain are heavily entangled; label one half of the chain A and the other half B. You can have a fair indication of what to expect when measuring the other half of the spins by measuring half of them first.
Then, they shifted measurement from the process’s finish, when the particle chain had already attained a certain quantum state, to the process’ beginning, when entanglement was just beginning to propagate. Because entanglement and measurement are mortal enemies, doing so resulted in a dispute. A collection of particles’ quantum state, when unaltered, contains all potential combinations of ups and downs that could result from measuring those particles. But a measurement causes a quantum state to collapse and eliminate any entanglement it may have had. Any alternative options are lost and you receive what is received.